7.1 – Discrete energy and radioactivity
Discrete Energy Levels
Electrons occupy different orbits around the nucleus at discrete energy levels. These energy levels are quantized, meaning that only certain values of energy are allowed, and there are gaps between energy levels that cannot be occupied by electrons.
For an electron to jump between energy levels, it must either gain or lose energy in the form of absorbing or releasing a photon. The energy of the photon will equal to the energy difference of the two energy levels.
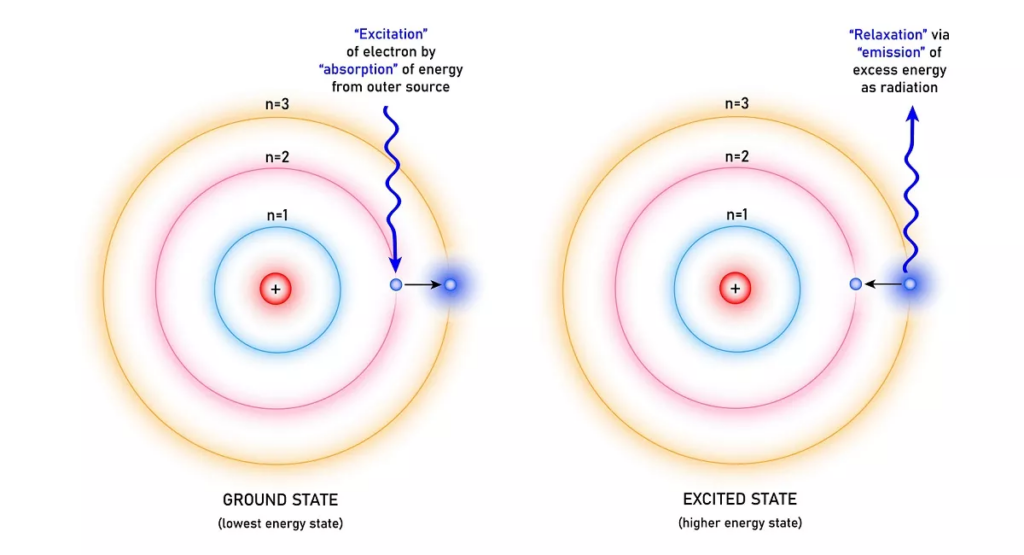
The photon’s energy is directly related to its frequency, given by E = h*f, where h is plank’s constant. The higher the energy of a photon, the higher its frequency.
Every atom has different energy levels, which makes it possible to identify an atom through an absorption or emission spectrum.
The wavelengths that are absorbed and emitted correspond to the different energy levels of the atom, like a ‘fingerprint’ that is unique for every element on the periodic table.
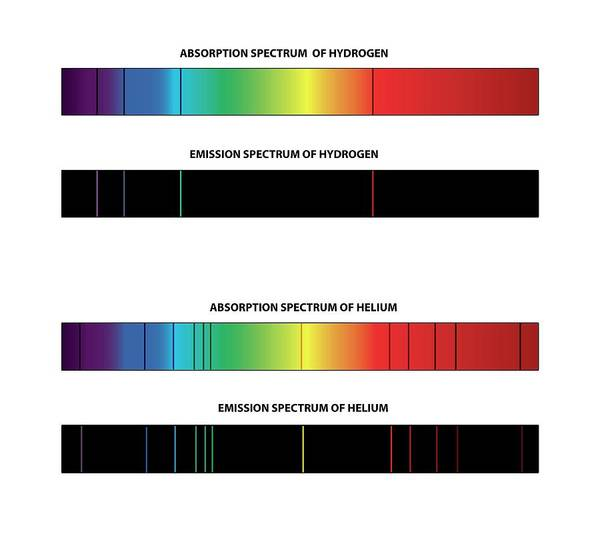
To calculate the wavelength of the photon, we use the following formula (also in your formula booklet):
λ=hcE
Radioactive decay
A spontaneous and random process where an unstable nucleus emits a particle in order to become more stable.
Can emit Alpha particles, Beta particles or Gamma rays.
The product from radioactive decay is the daughter nucleus, plus the decay particle that is emitted.
The exact moment for decay to occur in one nucleus is completely random, but when you have a large sample of nuclei, their probability of decay is highly predictable.
The probability of decay can be measured in terms of half-life.
Half-Life
Half-life is the time it takes for half of the radioactive sample to decay. If we have a sample with 10 nuclei, and it takes 2 seconds for 5 of them to have decayed, then we say the half-life is 2 seconds.
Some nuclei have very short half-lifes, and others are very, very long. Uranium-238 has a half-life of 4.5 billion years, while phosphorus-30 is two and a half minutes.
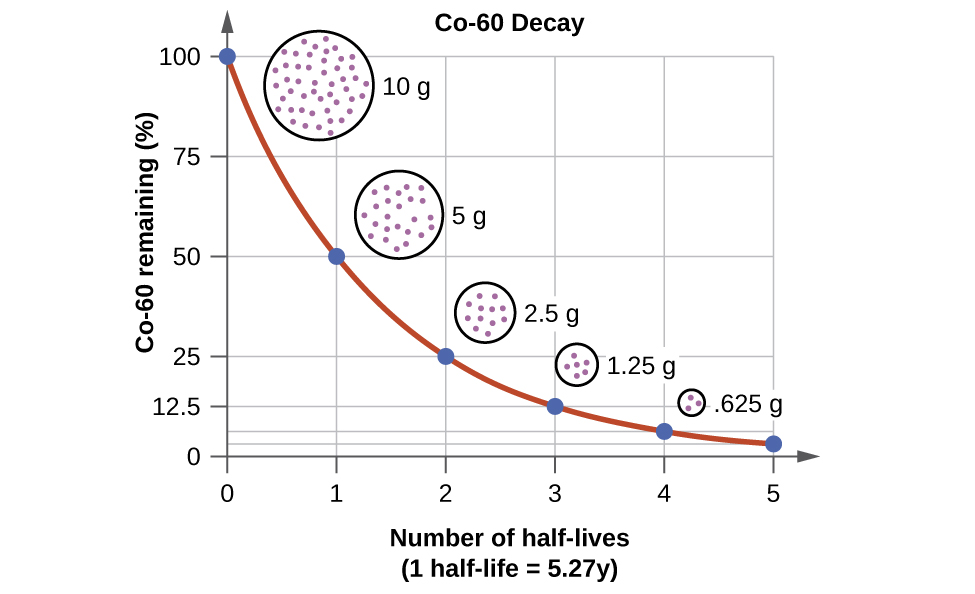
Types of Radioactive Decay
Mass Number (A) = total number of protons and neutrons
Atomic Number (Z) = number of protons
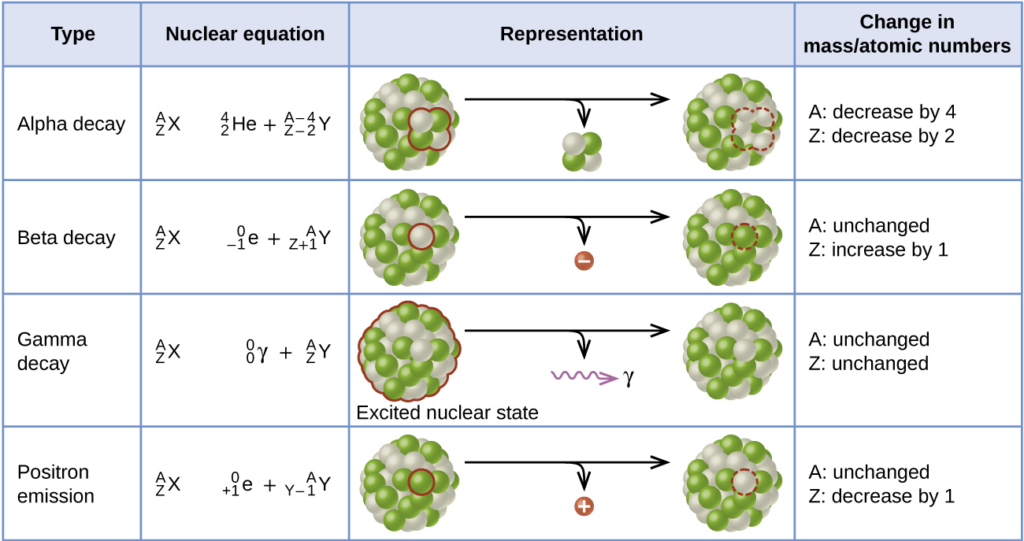
Alpha (α) Decay:
When a part of the nucleus is emitted in the form of a helium nucleus (alpha particle). An alpha particle is just a Helium nucleus and has a 2+ charge. In an alpha decay, the alpha particle is emitted, decreasing the number of protons and neutrons in the unstable nucleus.
Beta-minus (β-) Decay:
A beta (β) particle is simply an electron. When a radioactive nucleus has more neutrons than protons, one of the neutrons can break off into a proton and an electron, along with an antineutrino. The daughter nucleus gains a proton, and the excess electron is emitted.
Beta-plus (β+) Decay / Positron Emission:
A positron is identical to the electron, except with an opposite, positive charge. When the radioactive nucleus has more protons than neutrons, a proton breaks off into a neutron and a positron, along with a neutrino.
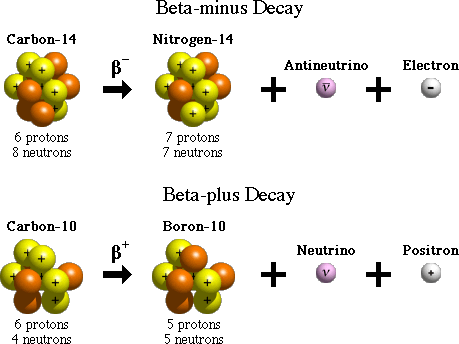
Gamma ray (γ) emission:
Gama rays are high-energy photons. They have no mass and no charge. The resulting nucleus will have the same number of protons and neutrons, but loses energy.
Absorption of Radiation
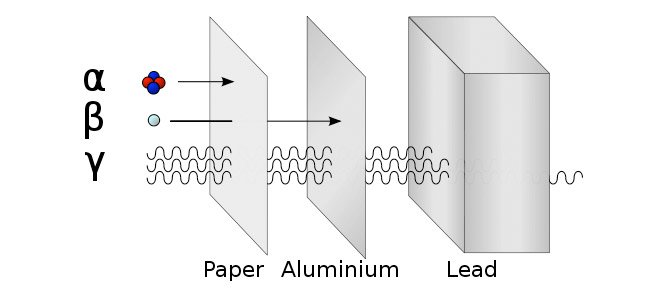
The way particles interact with materials depends on their ionizing ability. Alpha particles are the most ionizing and are easily absorbed by thin paper, and have a very short range in the air. This is because they are relatively massive with a 2+ charge, which makes them interact strongly with matter.
Beta particles are less ionizing but can travel longer in the air and need a thick sheet of aluminium to be absorbed.
Gamma rays barely interact with anything, as they are photons with no mass or charge, so it takes several centimeters of lead to absorb gamma radiation.
Background Radiation
Prolonged exposure to high levels of radiation can be harmful. However, humans have evolved to tolerate small levels of background radiation, such as cosmic radiation, gas or rocks that emit radiation from the ground, and even trace amounts of radioactive isotopes inside our own bodies.
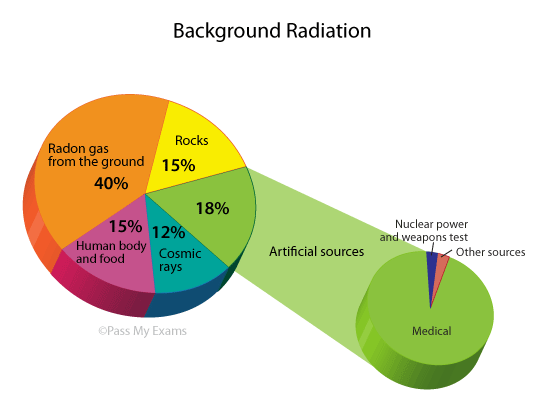
Isotopes
Isotopes are different ‘versions’ of the same element. They always have the same number of protons (thus they are the same element), but different number of neutrons. This difference in neutrons can make some isotopes more stable than others of the same element.
For example, carbon is an element with 6 protons. Carbon-12, the most common and stable isotope, has 6 neutrons. However, another natural isotope of carbon is Carbon-14, which has 8 neutrons.
Fundamental Forces
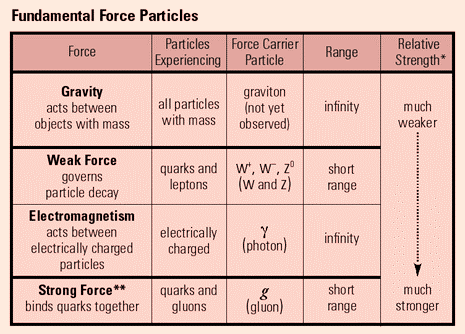
7.2 – Nuclear reactions
The Unified Atomic Mass Unit (u)
the mass of a Carbon-12 atom, roughly the mass of a proton or neutron. A unit used to measure masses in the atomic scale. It is equal to 1.661 × 10-27 kg.
Mass defect and nuclear binding energy
The nuclear binding energy is the amount of work required to break apart the nucleons in a nucleus held together by the strong force.
If you measured the mass of a single atom, its mass would be different than the sums of each nucleon inside that atom. This difference is called the mass defect.
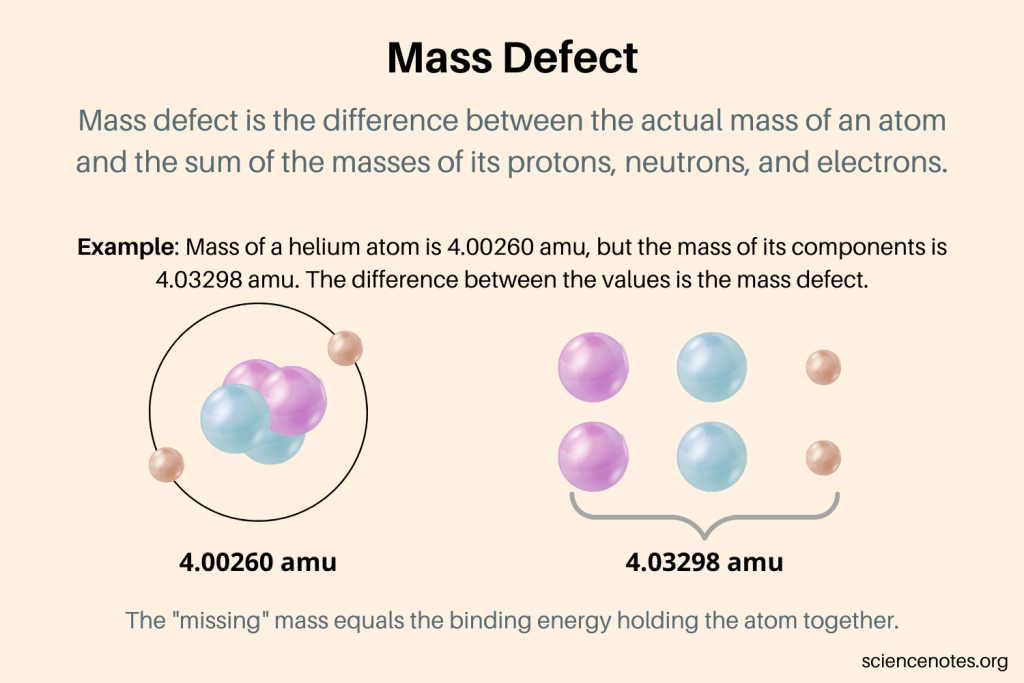
Mass defect can be explained by Einstein’s famous mass-energy equation, stating that mass and energy are interchangeable.
E=mc2
As energy is required to separate a nucleus, the total energy of the nucleus is higher than the total energy of the constituent particles. This difference in total energy creates the mass defect.
Nuclear fission and nuclear fusion
Nuclear Fission:
The reaction used in nuclear reactors and atomic bombs. A heavy nucleus (typically uranium) collides with a neutron and splits into two smaller nuclei, releasing more neutrons and energy.
The released neutrons continue to cause more reactions, this is a chain reaction.
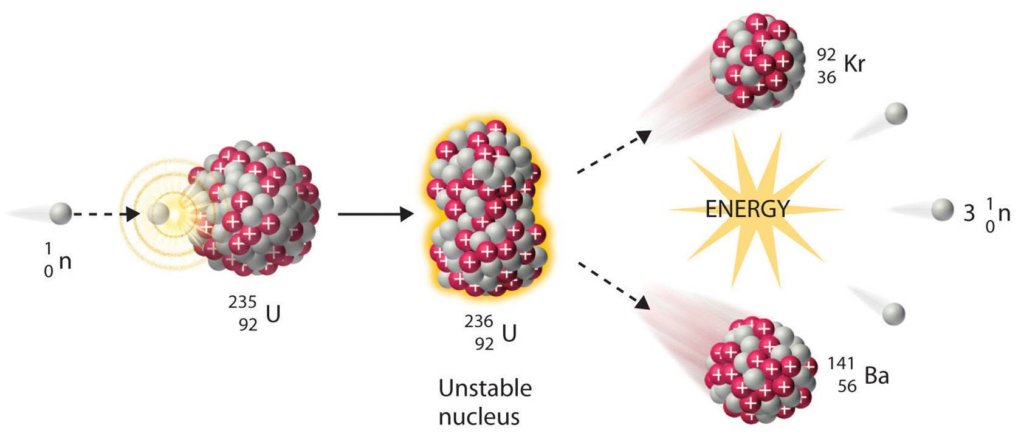
Nuclear Fusion:
Two smaller nuclei fuse to create a heavy nucleus, releasing energy in the process.
The source of energy for stars
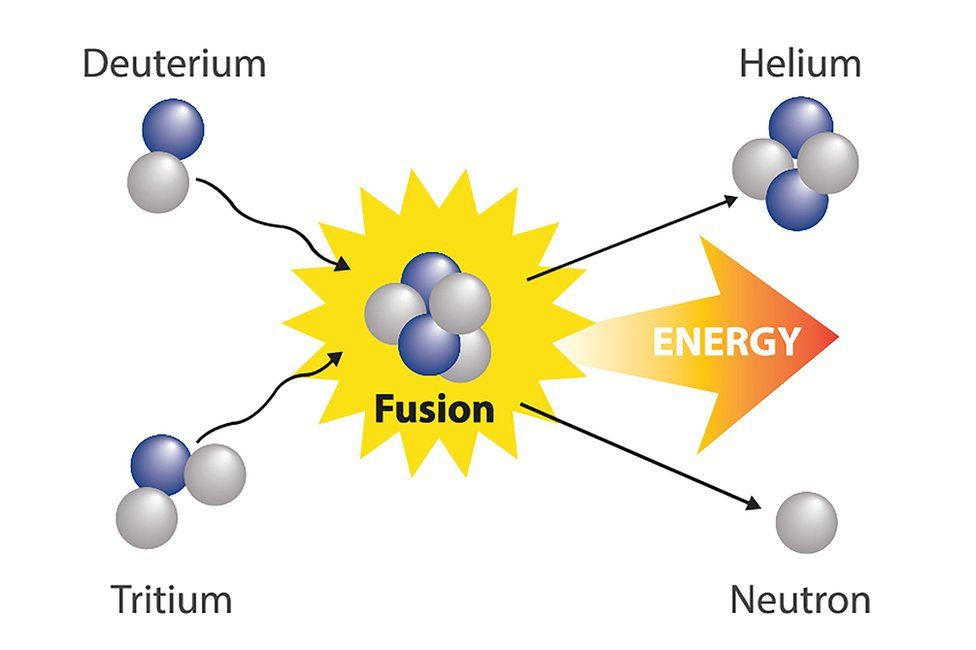
7.3 – The structure of matter
The Standard Model of Particle Physics
- It was previously believed that the smallest particles that existed were the ones that make up the atom (protons, neutrons, and electrons). We later discovered that these particles are actually made up of even smaller particles, called elementary particles.
- The most fundamental building blocks of matter are these elementary particles, namely fermions, which can be subdivided into quarks and leptons. Each particle has a constituent antiparticle.
- When these particles collide with their antiparticle, they ‘annihilate’ and release energy.
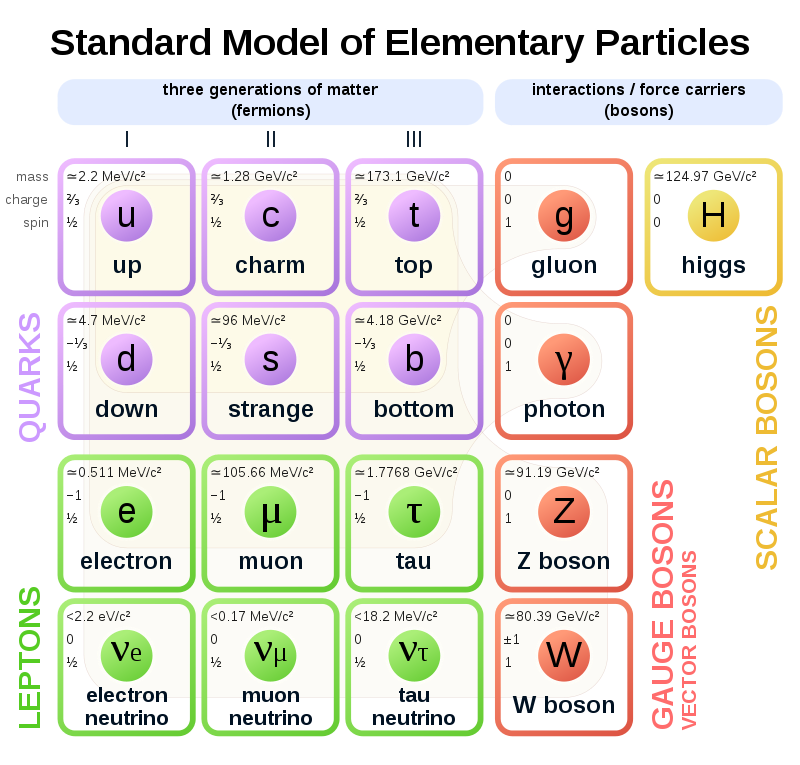
Quarks, leptons, and their antiparticles
Leptons
Leptons are part of the electron family. There are 6 leptons, the electron, electron neutrino, muon, muon neutrino, tau, and tau neutrino, each with corresponding antiparticles.
Quarks
Quarks are the fundamental building blocks of matter, they make up protons and neutrons, which together form an atom. There are 6 quarks; up, down, strange, charm, bottom and top, each with their antimatter counterpart.
Exchange particles
Exchange particles, also called bosons, are the way in which elementary particles interact with the four fundamental forces. These are gluons, photons, W+ bosons, W- bosons, Z0 bosons, and gravitons, and they each correspond to one of the four fundamental forces, shown in the graph below.
Hadrons, baryons, and mesons
- Hadrons are particles that are made up of quarks. Protons are made of up, up, down quarks and neutrons are made of up, down, down quarks.
- Hadrons can either be Baryons or Mesons. Baryons are made up of three quarks, or three antiquarks, but never a mix of both. Mesons are made up of a quark and antiquark. Protons and neutrons are classified as baryons as they are made up of three quarks.
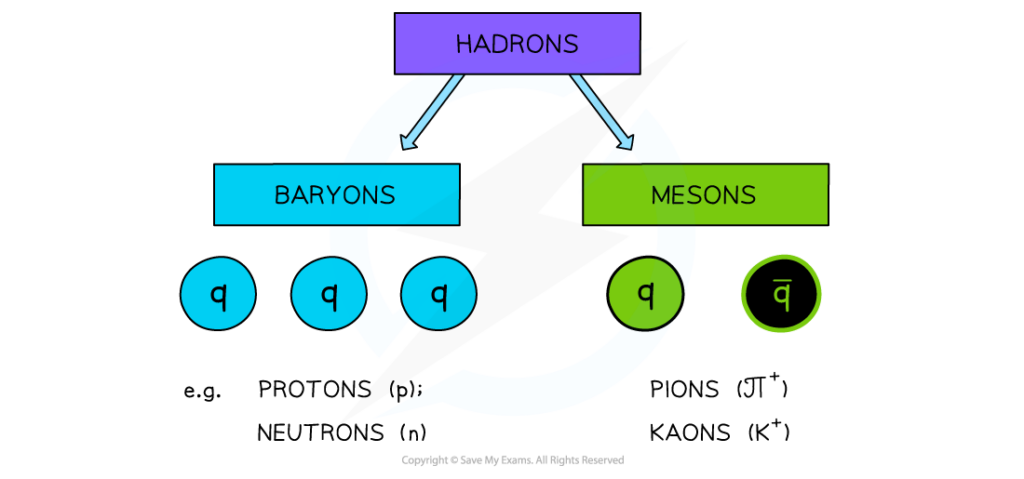
Quark Confinement
Quarks are held in place by the strong nuclear force (gluons) to form Hadrons. They are never found on their own.
Conservation Laws
In particle interactions and radioactive decay, charge, baryon number (B) and epton number (L) must be conserved. Only in strong interactions and electromagnetic interactions, strangeness is conserved.
- All leptons have a lepton number of +1, and antileptons have a lepton number of -1.
- All baryons have a baryon number of +1, antibaryons have a baryon number of -1.
Feynmann Diagrams
Feynmann diagrams were proposed by the famous physicist Richard Feynmann, as a convenient way to express particle interactions in time. The progression through time is read from left to write, where the x axis is time, and the y axis shows the direction of the particles.
Some diagrams switch the x and y axes, but it will be made clear which one is the time axis.
The Higgs Boson
In addition to the force carrier bosons, there is an additional, massive boson called the Higgs boson. Named after Peter Higgs, who proposed the existence of the Higgs boson in 1964 to explain how particles acquire mass, the particle was later discovered by CERN in 2012.